ION Science explains Photo-Ionization Detectors in ‘What is a PID?’
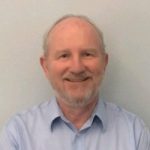
This guest blog, contributed by our partners at ION Science, takes a deep dive into the technical aspects of photoionization detection (PID). While it's more technical than our usual content, it's packed with valuable information for those looking to understand how PID technology works and where it applies. If you’re seeking a detailed breakdown of what a PID is, how it functions, and why it matters in gas detection, this article will provide all the insight you need.
ION Science explains Photo-Ionization Detectors in ‘What is a PID?’
PID is the abbreviation for “Photo-Ionization Detector”. A PID is a hand-held, personal, or fixed wall-mounted detector that measures a broad range of volatile organic compounds (VOCs) and some inorganic compounds in the parts-per-million (ppm) to parts-per-billion (ppb) range. It gives a continuous read-out and can alarm when concentrations exceed user-defined set points. It can also log data, calculate Time-Weighted Average (TWA) and Short-Term Exposure Limit (STEL), and alarm above these hygiene values. Advanced models use an internal pump to draw the air sample in from distances up to about 30 m. PIDs are most commonly used for industrial hygiene measurements to ensure that workers are not overexposed to toxic compounds, along with numerous secondary uses:
- fuel vapors
- solvents
- paint booths
- chemical spills
- confined space entry
- indoor air quality
- soil sample screening
- environmental clean-ups
- leak detection
- fenceline monitoring
- LEL measurements
- chemical process control
- heat transfer fluids
- cleanlabs
- arson investigation
- and many more
How a PID Works
Figure 1 is a schematic of an Ion Science sensor at the heart of the Tiger. A UV lamp generates high-energy photons, which pass through the lamp window and a mesh electrode into the sensor chamber. Sample gas is pumped over the sensor, and about 1% of it diffuses through a porous membrane filter into the other side of the sensor chamber. The inset on the lower right of Figure 1 shows what happens on a molecular level when a photon with enough energy strikes a molecule M, an electron (e-) is ejected. The M+ ion travels to the cathodic electrode, and the electron travels to the anode, resulting in a current proportional to the gas concentration. The electrical current is amplified and displayed as a ppm or ppb concentration. Not all molecules can be ionized. Thus, the major components of air, i.e., nitrogen, oxygen, carbon dioxide, argon, etc., do not cause a response, but most VOCs do give a response.
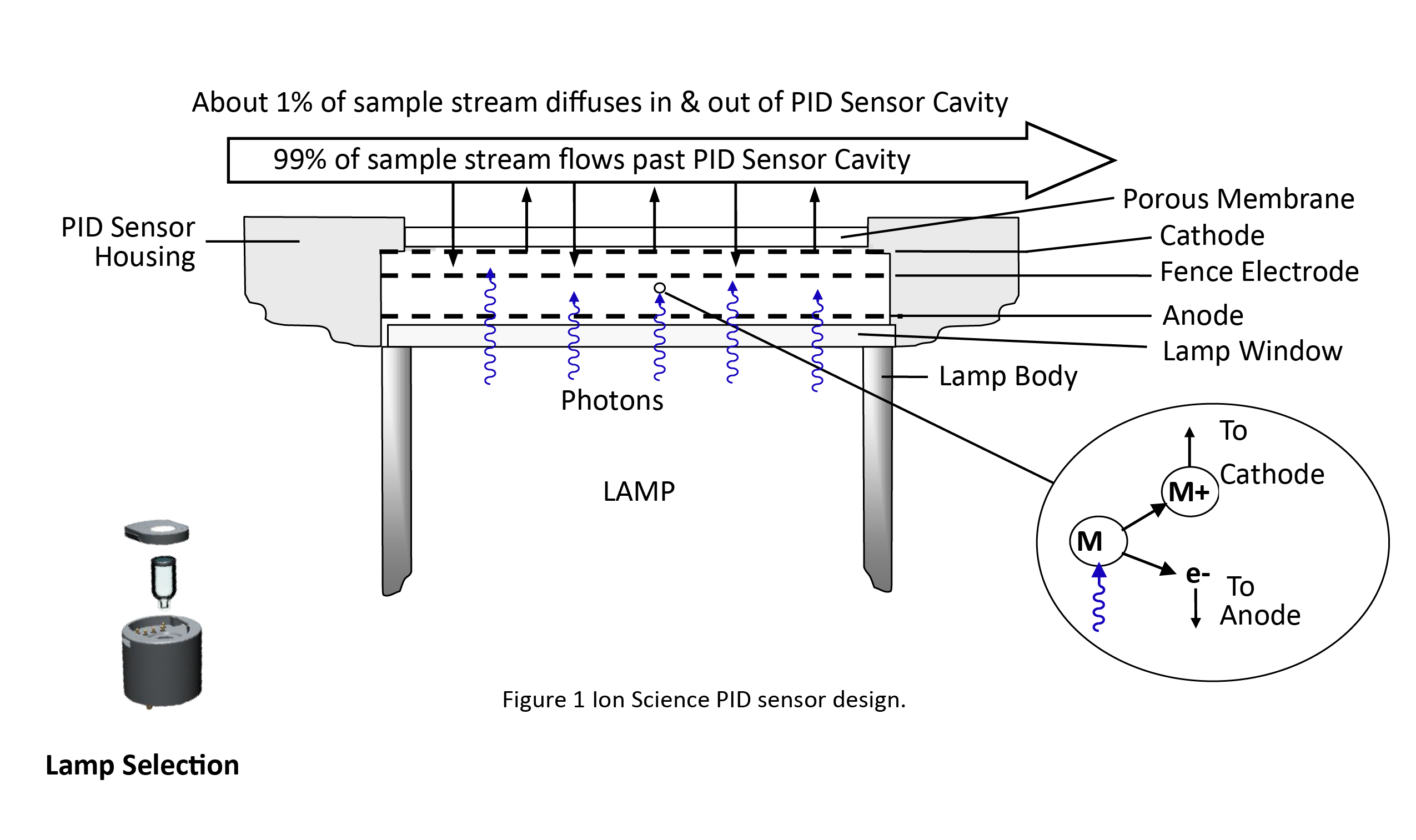
Lamp Selection
Three PID lamps are available with maximum photon energies of 10.0 eV, 10.6 eV, and 11.7 eV. Figure 2 illustrates that a lamp can only detect those compounds with ionization energies (IE) equal to or below that of the lamp photons. Thus, a 10.6 eV lamp can measure hydrogen sulfide with IE of 10.5 eV and all compounds with lower ionization energy but cannot detect methanol or compounds with higher IE.
The choice of lamp depends on the application. When only one compound is present, one can use any lamp with enough photon energy, often the standard 10.6 eV lamp, which has the lowest cost and has a long working life of up to a few years. For high IE compounds like chloroform, it is necessary to use the 11.7 eV lamp, which has a short life of only a few months. In the case of compound mixtures, use the lowest energy lamp possible. For example, to measure acetone in the presence of isopropanol, one could use the 10.0 eV lamp, which does not interfere with isopropanol.
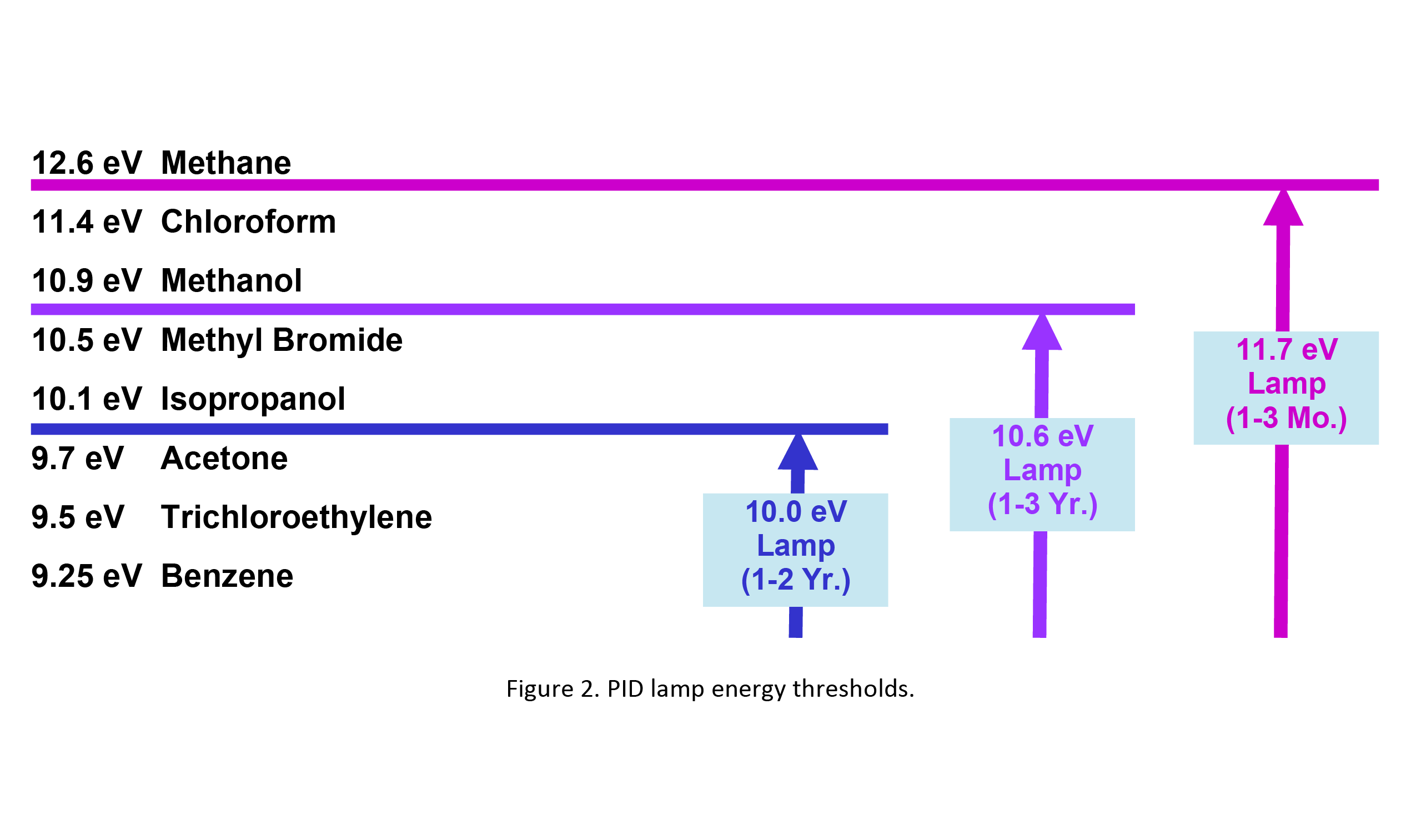
What Compounds a PID Can Measure
PIDs can detect many thousands of VOCs. The sensitivity is roughly in the following decreasing order:
- Aromatics, like benzene, toluene, xylene, pyridine, phenol, aniline, aphthalene
- Olefins, like butadiene, cyclohexene, trichloroethylene, vinyl chloride, turpentine, limonene cleaner
- Bromides & Iodides, like methyl bromide fumigant, n-bromopropane degreaser, iodine disinfectant
- Sulfides & Mercaptans, like methyl mercaptan natural gas odorant
- Organic Amines, like methylamine, trimethylamine (prone to unstable response)
- Ketones, like acetone, methyl ethyl ketone (MEK), methyl isobutyl ketone (MIBK)
In addition, there is varying response to chlorinated compounds, organic acids, silicate-, borate-, & phosphate- esters, isocyanates, and many others. Fuels like automotive gasoline (petrol), diesel, kerosene, and jet fuels are mixtures of aromatics, olefins, and alkanes, and thus, they give a strong response.
What a PID Does Not Measure
There is no response to:
- Components of Clean Air, like nitrogen, oxygen, carbon dioxide, water vapour, argon
- Noble Gases, like helium, xenon, krypton, argon
- Most Small Molecules, like hydrogen, carbon monoxide, HCN, ozone, hydrogen peroxide, SO2
- Natural Gas, including methane and ethane
- Mineral Acids, like hydrochloric acid, nitric acid, sulfuric acid
- Fluorinated Compounds, like Freon® refrigerants, anaesthetic gases, sulfur hexafluoride
- Non-Volatiles, like PCBs, PAHs, MDI, greases, waxes
- Radioactives, like uranium, plutonium, radon
Programmed Response Factors
TA-02 (Ion Science PID Response Factors) quantitates the sensitivity of over 900 compounds in the form of response factors (RFs) relative to the response of isobutylene. Although it is most accurate to calibrate with the compound to be measured, such calibration gas is often not readily available, making calibration with standard isobutylene gas very convenient.
When a PID is calibrated with isobutylene, the readings of other compounds need to be converted, as follows:
True concentration = PID Reading x RF
Table 1 gives some RF values for a few chemicals. For example, if a PID calibrated with isobutylene is used to measure benzene with a 10.6 eV lamp and the reading is 10 ppm, the true concentration is:
Benzene concentration = 10 ppm x 0.50 = 5.0 ppm benzene
If the same result is obtained with ethanol, the true concentration is:
Ethanol concentration = 10 ppm x 11 = 110 ppm Ethanol
Table 1. Response Factors of Example Compounds with PID Lamps
Note that RFs are inverse to sensitivity, i.e., the lower the RF, the more sensitive the compound. It takes 110 ppm of ethanol to give the same 10 ppm response as 5.0 ppm benzene.
Pre-Programmed Response Factors
Most Ion Science PIDs come pre-loaded with 800+ RFs that can be called up by the user. In that case, the display gives the concentration of the chemical directly, and no extra calculations need to be done. Note that calling up an RF from memory does not make the PID more selective for that particular chemical – it still responds to all detectable chemicals present if there is a mixture.
Patented Fence Electrode Prevents Humidity Problems
Most PIDs suffer from low readings when humidity is high due to quenching by water vapour, as shown by the green triangles in Figure 3.
The opposite, i.e., drifting high readings at high RH, can also occur when the sensor becomes contaminated. Ion Science has solved these two issues with a combination of a fence electrode and an anti-contamination system design (see Figure 1), whereby the sensor is protected by diffusion of the gas through a filter. Other manufacturers attempt to compensate for the RH quenching by adding a humidity sensor.
However, as shown by the brown squares in Figure 3, compensation is often inaccurate and can overcompensate, giving false high readings. By contrast, the Ion Science sensor (blue diamonds in Fig. 3) is not affected by humidity and requires no artificial compensation and thus is much more reliable and accurate in normal humid air. This feature makes Ion Science PIDs especially useful for such applications as soil headspace analysis during environmental clean-ups, where the samples are both highly humid and prone to dirt and dust contamination, which create severe problems for other PIDs.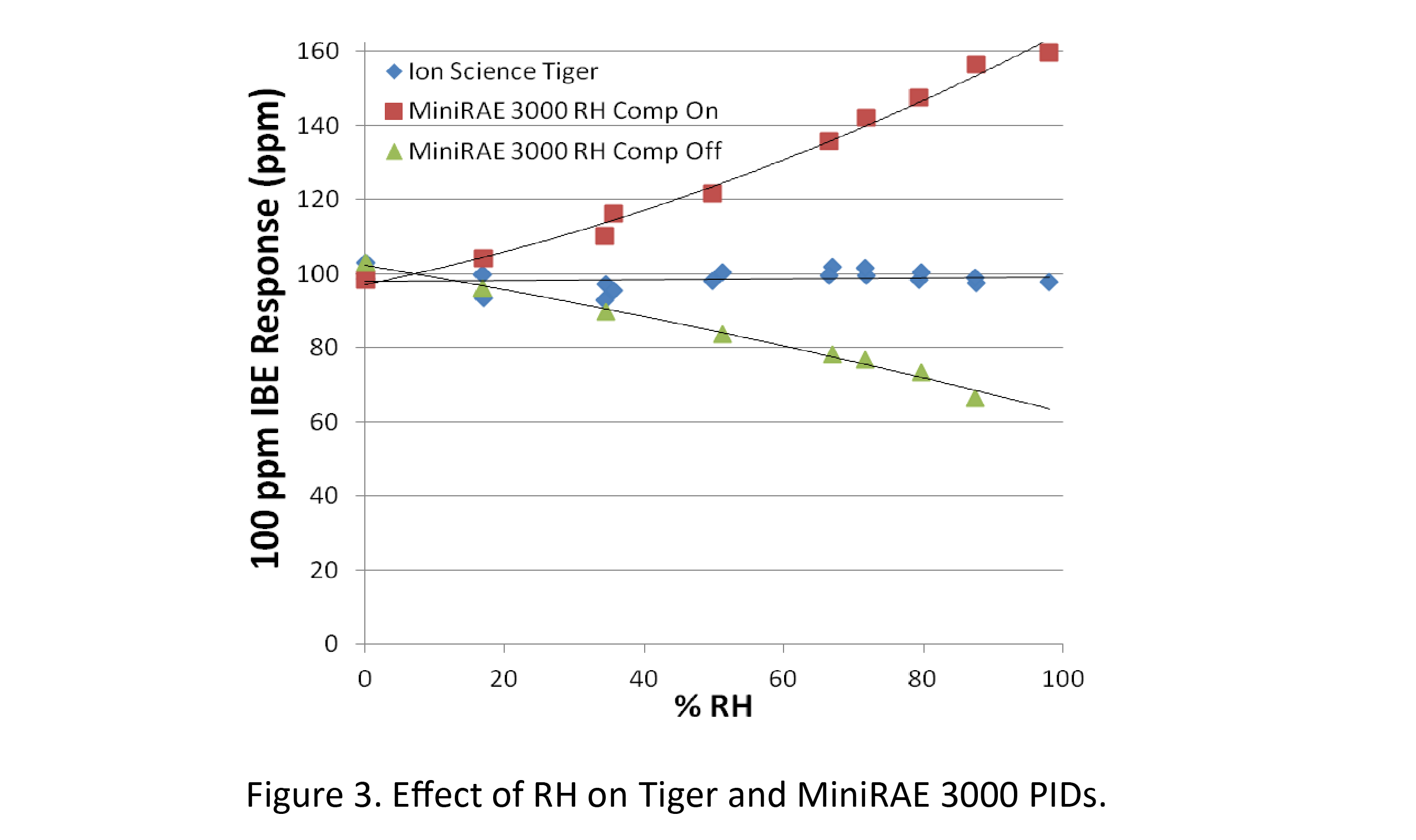
For more details on PID humidity effects and the function of the fence electrode, see TA-06 (Patented Fence Electrode Eliminates Humidity Effects on Ion Science PIDs).
PIDs for LEL Testing
PIDs are useful for measuring the flammability of an atmosphere. Measurement of vapour flammability, or LEL (lower explosive limit), is usually done with a pellistor or catalytic bead sensor. Commonly, the alarm is set at 10% of LEL to ensure a good margin of safety. The LEL for many VOCs is a few Vol %. Since 1% Vol equals 10,000 ppm, 10% of LEL is typically in the range of several 100 to a few 1000 ppm, which is an easy range for a PID. For example, styrene and vinyl chloride have LELs of 1.1 Vol % and 4.0 Vol %, for which 10% LEL equals 1100 ppm and 4000 ppm, respectively. LEL sensors are lower cost than PIDs but have drawbacks:
- Weak response to heavy hydrocarbons like diesel & jet fuels, turpentine, e
- Are positioned by a) polymerizable compounds like styrene & vinyl chloride, b) silicones, and compounds containing sulfur, chlorine, bromine, phosphorus, lead, etc.
PIDs do not suffer from these problems and thus are useful for measuring LEL in these situations. Examples include;
- airlines relying on PIDs for LEL safety during wingtank entry (jet fuel),
- manufacturing plants using silicone lubricants
- deodorant filling plant, and polystyrene manufacturing and curing.
PIDs Do Not Require Oxygen
PIDs can function in cases where it is necessary to measure toxic compounds in the absence of oxygen. By contrast, most electrochemical sensors and pellistor-type LEL sensors require oxygen to function. Even though workers use supplied air in such situations, it may be necessary to measure VOCs because of skin exposure hazards or the potential for explosion should air suddenly intrude. Some chemical processes control situations also are run in inerted atmospheres and thus can use a PID to monitor VOCs.